Speeding the Adoption of Additive Mfg.
Ashley Eckhoff | Industrial Equipment News
Speeding the Adoption of Additive Mfg.
When beginning to consider all that is needed to make additive a reality, it becomes clear that traditional ways of designing and manufacturing parts are falling away.
Additive manufacturing (AM), or 3D printing offers a number of potential innovations in product design, while its flexible manufacturing capabilities can support a distributed manufacturing model - helping to unlock new business potential. However, when companies begin to consider all that is needed to make additive a reality— such as generative design, part consolidation, and topology optimization—it becomes clear that the traditional ways of designing and manufacturing parts are falling away.
However, adoption of new manufacturing technologies always faces challenges. Fortunately, great strides have been made in bringing AM into the mainstream. Emerging tools, processes and technologies have come to the fore that are moving adopters closer to industrialized additive, most notably in the form of simulation.
A Brave New Material World
Material choice is the first decision and one of the most important for AM applications. The selection is based on design requirements, including weight, strength, thermal performance and cost targets. For example, a designer might choose titanium to meet specific strength parameters, whereas choosing aluminum meets cost constraints. Both materials are printable using AM techniques, but the choice of materials depends on the design and business needs.
Presently, the story of materials for additive manufacturing is one of growing potential—both for metals and polymers. The list of usable materials does not compare with those available for traditional manufacturing methods, but it is growing. In the past five years, certified AM materials have expanded from several hundred to over 3000, with new ones being continuously certified. We expect to see the market for printable materials continue to grow, and we are excited by the new design possibilities that expansion will enable.
Advanced simulation and CAD capabilities will be essential in fully understanding these new material properties during the design and validation of an AM part. Simulation using conventional, standard FEA techniques cannot simulate these materials without extensive up-front calibration.
Recently, new multiscale FEA analysis capabilities have emerged that help predict a 3D printed part’s durability by combining a local scale model of the microstructure with a global scale model of the part being analyzed. These two analyses are performed simultaneously, and the results from one model affect the behavior of the other. This method of solving each domain individually and then linking the results has been shown to be as more accurate than the standard FEA technique, with 1,000x the efficiency. The result is the ability to simulate the complete performance of materials in printed parts.
Innovative Geometries
Additively manufactured objects can be nearly any shape or geometry as defined by the 3D model. It is a geometry agnostic technology, allowing companies to manufacture and produce intricate geometries previously impossible with traditional injection molding or subtractive manufacturing methods. The near-limitless geometries also enable part consolidation.
Instead of breaking a system into multiple components for manufacturability with CNC or casting methods, AM can reduce even the most complex geometries into a few or even one part. The savvier engineer may even integrate functional capabilities to the system like springs or mechanical switches to further reduce part counts.
These complex geometries can be a double-edged sword, as they are not easily defined in traditional CAD techniques. Tools supporting multi-disciplinary generative engineering with powerful simulation capabilities are required. Design space exploration capabilities can automate the process of finding optimal designs that meet a multiplicity of performance requirements, iterating on hundreds of possible designs in a matter of minutes. And it helps users visualize design performance trade-offs between competing objectives and constraints.
Simulation is the friend of every designer working with AM technologies. When designing a part for additive manufacturing, it is imperative to verify and simulate the print before committing to manufacturing. The medical industry is very familiar with this, being an early adopter of 3D printing to create custom implants such as replacement joints.
As part of this process, designers rely on specialized tools for simulating the metal printing process. Printing a hip joint in titanium can take up to 32 hours; a problem occurring mid-way through a print could cost up to $50K in wasted time and materials. Therefore, simulation before physically printing can save time and money.
Scaling for Industrial
Another hurdle for additive manufacturing is scaling it to mainstream production, producing thousands of parts quickly with high quality. While progress has been made towards this goal, there are still unknowns and challenges for each processing method. Whether it is thermoplastics, composites or metal, there are many material interactions still not fully understood. This is a problem for part repeatability and process accuracy, creating a need for new design methods and new technology.
Additive manufacturing involves heating materials to extreme temperatures and then cooling the material into the desired shape. Printed parts cool in relative free-space. Consequently, the parts do not cool homogeneously, which can lead to unexpected distortions. As a result, numerous iterations are often required to attain acceptable thermal loading and cooling cycles, leading to significant scrap and often defeating the purpose of utilizing AM’s rapid production capabilities.
Fortunately, robust simulation based on computational fluid dynamics (CFD) techniques can predict thermo-mechanical distortions and then propose possible alterations to the CAD geometry to rectify those distortions. In addition, advanced AM build preparation and simulation software are available to predict and correct mesoscale defects from over-heating as the extruder or laser moves along its 3D printing path.
It is also possible to predict where and why the part might fail, helping designers to optimize 3D printed parts down to the microstructural level. As these solutions for driving sophisticated 3D printing machinery, achieving a repeatable, quality, industrial AM process will become commonplace.
Maximizing the Potential
Even in this brief discussion, it is evident that tackling AM challenges requires adopting a new mindset for design and a new approach to manufacturing. For most early adopters, this is a gradual process as opposed to an all-or-nothing approach.
Often, this process starts at a minimal level. In Germany, automotive companies experimenting with a combination approach are using additive manufacturing and design software to create structurally sound pieces of a car frame. They may not be printing an entire car, but they are taking extruded hollow beams, like those used in traditional automobile manufacturing, and printing nodes to connect those beams together.
These nodes include a printed lattice structure on the interior to generate the required structural rigidity with a minimal weight penalty. By starting small and focusing on an achievable goal, they’re printing durable, efficient parts that could eventually change future automobile manufacturing.
Meanwhile in the aerospace industry, quality production is being augmented by sophisticated simulation. Simulation-driven design techniques are now utilized to minimize part weight and material usage while maintaining necessary structural characteristics. Then, during manufacturing, simulation is used again to ensure a quality output from the build setup and print process.
In examples like these we see technology augmenting production across industries and across the world. This is the type of innovation and evolution of additive manufacturing that will be required to bring the technology to industrial production levels.
Additive manufacturing may be relatively innovative, but it is rapidly growing as new materials are certified, generative engineering is embraced, topology optimization becomes the norm and manufacturing software is introduced and adopted. Traditional manufacturing methods have had centuries to become established: casting has been around since the Bronze Age and forging since the Iron Age.
We are only beginning to learn how AM technology can change design and manufacturing. During this exciting time of discovery and maturation, a design ethos focused on AM’s abilities and challenges and a new approach to manufacturing is moving AM closer to sparking the next industrial revolution.

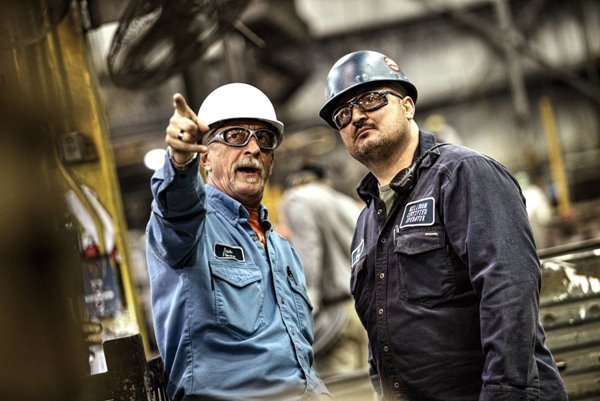